Algae are tiny
biological factories that use photosynthesis to transform carbon dioxide and
sunlight into energy so efficiently that they can double their weight several
times a day. Algae oil is an interesting sustainable feedstock for biodiesel
manufacturing. It is an alternative to popular feedstocks, like soybean, canola
and palm. In comparison to traditional oil-seed crops, algae yields much more
oil per acre. While soybean typically produces less than 50 gallon of oil per
acre and rapeseed generates less than 130 gallon per acre, algae can yield up
to 10,000 gallons per acre. In particular diatoms and green algae are good
sources for the production of biodiesel.
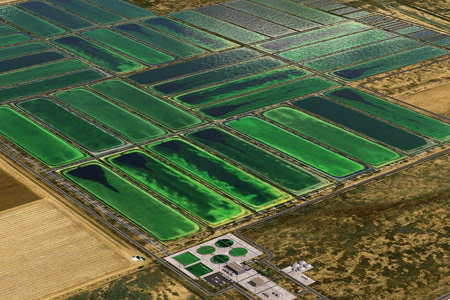
History as Fuel
The idea of using algae
as a source of food, feed and energy goes back more than half a century.
Production of methane gas from algae was proposed in the early 1950s, and
received a big impetus during the energy crisis of the 1970s, when projects
were initiated to produce gaseous fuels (hydrogen and methane). From 1980 to
1996 the US Department of Energy supported the Aquatic Species Program (ASP), a
relatively small effort (about $25 million over almost 20 years) with the
specific goal of producing oil from microalgae.
So exactly how can we get
oil from algae?
Algae are grown in either open-pond or
closed-pond systems. Once the algae are harvested, the lipids, or oils, are
extracted from the walls of the algae cells.There are a few different ways to
extract the oil from algae. The oil press is the simplest and most popular
method. It's similar to the concept of the olive press. It can extract up to 75
percent of the oil from the algae being pressed.
Basically a two-part
process, the hexane solvent method (combined with pressing the algae) extracts
up to 95 percent of oil from algae. First, the press squeezes out the oil.
Then, leftover algae is mixed with hexane, filtered and cleaned so there's no
chemical left in the oil.
The supercritical
fluids method extracts up to 100 percent of the oil from algae. Carbon dioxide
acts as the supercritical fluid . when a substance is pressurized and heated to
change its composition into a liquid as well as a gas. At this point, carbon
dioxide is mixed with the algae. When they're combined, the carbon dioxide
turns the algae completely into oil. The additional equipment and work make
this method a less popular option.
Once the oil's
extracted, it's refined using fatty acid chains in a process called
transesterification. Here, a catalyst such as sodium hydroxide is mixed in with
an alcohol such as methanol. This creates a biodiesel fuel combined with a
glycerol. The mixture is refined to remove the glycerol. The final product is
algae biodiesel fuel.The process of extracting oil from the algae is universal,
but companies producing algae biodiesel are using diverse methods to grow
enough algae to produce large amounts of oil.




Different Bio fuels
made with algae
Biodiesel - Microalgae
are involved in the production of biodiesel. Microalgae are chosen for the production of
biofuel based on their oil content. The oil inside the microalgae is removed from algae by
chemical means or either squeezed out. The oil is collected and changed chemically and
is then used as an ingredient in biodiesel.
Bioethanol – Bioethanol
is an ingredient used in the production of petrol. Macroalgae that contain a lot of
sugar are chosen. Macroalgae are cut, mashed and treated.The macroalgae now
appear as sludge and is called feedstock. Other micro-organisms
called Yeast are added at this stage. Yeast uses the feedstock
as food and breaks down
the feedstock into ethanol and other components. This process is called fermentation.
Ethanol is separated from the other components and then the ethanol is used in
petrol.
Biogas – Methane is the
main ingredient in the production of biogas. Methane gas can be produced by using
macroalgae. The macroalgae must have a high sugar content. Macroalgae are cut and
mashed. Micro-organisms convert the natural sugars in macroalgae into
glucose. The macroalgae now appear as sludge and is called feedstock. The feedstock will go
into a big tank and special micro-organisms called anaerobes are
added. These
micro-organisms are special because they don’t need oxygen to survive.They work without
oxygen(air). They also need an environment that has the correct temperature and
acidity. When conditions are correct the special micro-organisms perform a series of
reactions called anaerobic digestion.Within this environment
methane and other gases are produced. The methane gas is separated and used as
biogas.
Hartman, Eviana (6 January 2008). "A Promising Oil Alternative: Algae Energy". The Washington Post. Retrieved 1 May 2014.
http://en.wikipedia.org/wiki/Algae_fuel